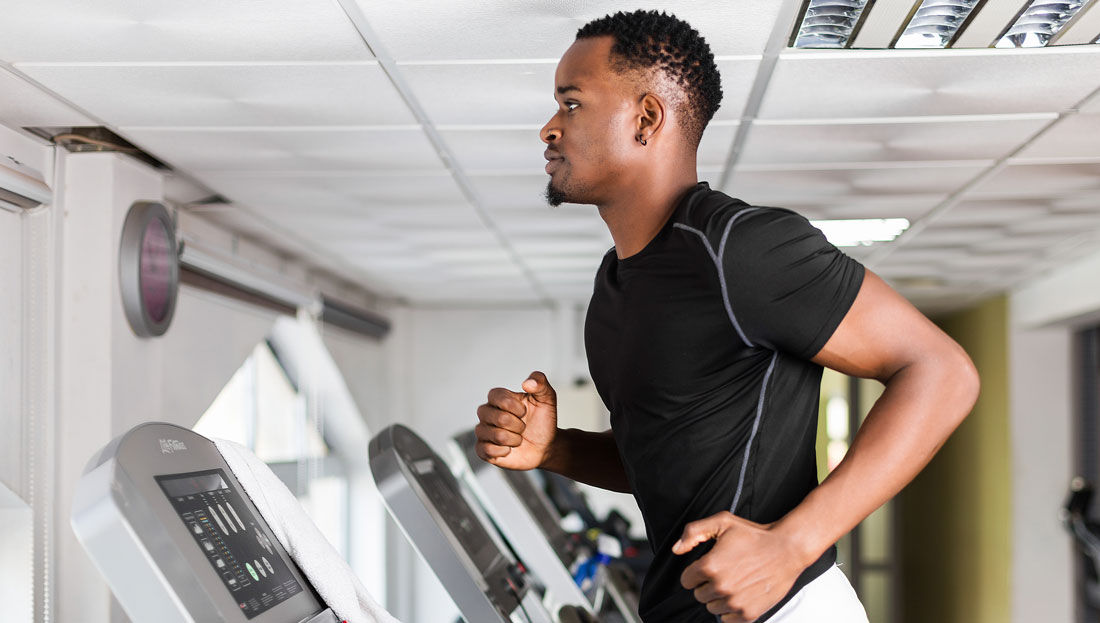
By Lance C. Dalleck and Gary P. Van Guilder
The Cardiorespiratory Training component of the ACE Integrated Fitness Training® (ACE IFT®) Model is founded on the premise of threshold-based programming. Over the past five years, a series of ACE-supported research studies has provided robust evidence that a threshold-based approach helps to personalize workouts for each client’s unique health, fitness and goals. This article explores how thresholds can be detected, including with wearable technologies, and explains how you can optimize training responsiveness with threshold-based programming.
The ACE IFT Model for Cardiorespiratory Training
The ACE IFT Model provides a systematic approach to cardiorespiratory training that can take a client all the way from being previously physically inactive to training for a personal record in an event such as a half-marathon. While this is not the training goal of most physically inactive individuals, having an organized system of training that allows for long-term progression is empowering because it provides you with strategies for training the entire spectrum of clientele—from the physically inactive person to the competitive athlete.
The Cardiorespiratory Training component of the ACE IFT Model is divided into three phases, each with a title that defines its training focus:
- Base Training
- Fitness Training
- Performance Training
The Cardiorespiratory Training component of the ACE IFT Model is founded on the premise of threshold-based programming and based on the three-zone intensity model using heart rate (HR) at the first and second ventilatory thresholds (VT1 and VT2) to develop personalized programs based on each client’s metabolic responses to exercise (Figure 1).
Figure 1. Three-zone intensity model using metabolic markers and the talk test
The Scientific Evidence for Threshold-based Training
It is well established that regular exercise has positive effects on health outcomes related to fitness and cardiovascular health. However, evidence also suggests considerable individual variability in exercise-induced changes in cardiorespiratory fitness and common cardiometabolic risk factors, with some individuals experiencing either a nonresponse or adverse response (a response in an unfavorable direction) when exposed to regular exercise. To increase responsiveness, it has been suggested that a threshold-based model for establishing exercise intensity might better identify the lowest effective training stimulus for achieving comparable relative metabolic demands across individuals with varying fitness levels.
Over the past five years, our team of researchers in the High Altitude Exercise Physiology Program at Western Colorado University has performed a series of ACE-supported research studies focused on the effectiveness of the ACE IFT Model and its threshold-based programming approach at eliciting favorable training responses. Collectively, our findings conclusively demonstrate that a threshold-based approach to individualized exercise programming produces better improvements in cardiorespiratory fitness and cardiometabolic risk factors and eliminates almost entirely the odds for a nonresponse or adverse response.
The Physiology of Threshold-based Training
What is the physiology behind the success of training based on ventilatory responses? In simple terms, ventilation reflects the amount of air that is transported into and out of lungs each minute (termed minute ventilation). Minute ventilation increases during exercise because both the amount of air exchanged with each breath (tidal volume) and the breathing frequency increase. If exercise intensity continues to gradually increase, ventilation also increases in a linear manner. Increases in tidal volume contribute more to the rise in ventilation at low-intensity exercise, while increases in breathing rate contribute more to the increase in ventilation during high-intensity exercise.
At a more complex level, however, the changes in ventilation that accompany exercise are actually very precise indicators of the metabolic stress of exercising muscle. If the metabolic stress of exercising muscle changes, then ventilation also changes accordingly and shows deflection points at specific intensities.
Factors Contributing to VT1
At a certain exercise intensity, the metabolic activity of the cell begins to produce higher levels of carbon dioxide, which is a metabolic waste product released from working muscle as a result of the energy demands of exercise. The best way to eliminate this waste product is to remove it by exhalation after it has been transported to the lungs. Therefore, it is an advantage of the respiratory system to increase ventilation when carbon dioxide levels get too high. Specialized sensors located throughout the body sense the higher levels of carbon dioxide. In turn, signals from these sensors are relayed to the brain, which then send signals to the respiratory muscles so they can increase the depth of inspiration and breathing rate. As a result, ventilation increases more steeply, which can be seen at the first deflection point (Figure 2). The exercise intensity at this deflection point is VT1. Evidence suggests that the increase in ventilation associated with VT1 is due primarily to a greater depth of breathing rather than increases in breathing rate. Greater ventilation rates at VT1 helps to maintain a balance in carbon dioxide production with elimination.
Figure 2. Ventilatory effects during exercise
Factors Contributing to VT2
As exercise intensity continues to increase past VT1, the need to inspire more oxygen and to expire more carbon dioxide is met by further increases in ventilation. At high-intensity exercise, increased frequency of breathing is the main way that ventilation rises. Eventually, ventilation continues to increase and reaches a second deflection point, or VT2. Therefore, VT2 represents a further disproportionate elevation in ventilation relative to oxygen consumption. At this level of exercise, the working muscle produces substantial amounts of acid metabolites (known as hydrogen ions) that lower blood pH. In an attempt to control the acid build-up, blood buffers neutralize these ions. In doing so, however, greater amounts of carbon dioxide are produced. Consequently, the respiratory system is called upon to accelerate breathing frequency beyond VT1. Therefore, VT2 represents the point at which the respiratory system compensates for the elevated acidosis (which is why VT2 is often referred to as the respiratory compensation threshold).
Just like at VT1, specialized sensors communicate the acidity and excess carbon dioxide to the brain; however, these are relayed with greater urgency to the respiratory muscles. In addition, more sensors are recruited at VT2. For example, there are sensors in the muscle that pick up more vigorous contractions related to muscle length, tension and force. Other muscle receptors detect precise changes in muscle pH and ion levels. Finally, increased body temperature that accompanies high-intensity exercise stimulates temperature receptors in the brain to increase breathing rate even more at VT2 (see Figure 2).
In summary, many types of sensors throughout the body communicate changes in metabolic stress during exercise to the brain, with the result being ventilation thresholds denoted as VT1 and VT2. A deflection point in ventilation reflects the level of exercise intensity in which the cell reaches a specific threshold of metabolic stress. Programming exercise around these metabolic thresholds, therefore, has the advantage of producing greater physiological adaptations to training because it can reveal what is going on at the cellular level.
Wearable Technologies for Detection of Thresholds
A key factor underpinning the success of threshold-based training at eliciting positive training responsiveness is the accurate detection of individual ventilatory thresholds (i.e., VT1 and VT2) from graded, exercise testing. However, in real-world settings it is generally both challenging and cost-prohibitive for most people to have regular access to metabolic testing in an academic or performance laboratory. As such, alternative options for detecting VT1 and VT2 are necessary to be able to apply threshold-based training to a larger demographic of the population.
In the past decade, there has been an explosion in wearable technologies that can be used for guiding exercise training, including apps, smart watches, heart-rate monitors and other devices. A more recent addition to wearable technology options is the Tyme Wear smart shirt. Sensors within the smart shirt measure ventilation and communicate wirelessly with a smartphone app. The app guides users through a 15-minute fitness assessment that uses data from the smart shirt to assess an individual’s unique fitness level from their first and second ventilatory thresholds. The app further displays their fitness level results and provides workouts that are customized to each individual’s unique fitness level to ensure optimal fitness improvement while minimizing the risks associated with overtraining and undertraining, such as injury and reduced fitness, respectively.
Alternative Options for Detection of Thresholds
As a health and exercise professional, you likely need alternative options for identifying VT1 and VT2. Each of the following field assessments can be used to detect thresholds and guide training:
- Talk Test
- Submaximal Talk Test for VT1
- VT2 Threshold Assessment
Note: You can find more information on how to conduct these and other assessments in the ACE Answers section of the ACE website (login required).
Sample Threshold-Based Programming for Performance Training
Regardless of the particular phase a client is in, proper exercise program design should focus on creating the appropriate frequency, intensity and duration to fit the individual’s current health and fitness level, while also providing effective progressions to help the client safely achieve their goals. Program design based on ventilatory thresholds is an excellent method to optimize training for clients pursuing performance goals. The primary goal of performance training is to continue to build on moderate- and vigorous-intensity exercise and incorporate zone 3 intervals that progress clients to intensities at and above VT2 to achieve greater improvements in cardiorespiratory efficiency and anaerobic capacity, and to sustain exercise at or near VT2 for an extended period of time.
Once you have identified the heart rates that correspond to a client’s VT1 and VT2, you can establish a three-zone model that meets the performance goals of the client. Remember, the submaximal talk test for VT1 can provide you with the client’s HR at VT1 to use when designing programs for improving general fitness. In contrast, the higher-intensity VT2 threshold assessment makes it possible to establish the client’s HR at VT2 to incorporate in more advanced programming for clients who have advanced-fitness and endurance sports–performance goals. It is important to note that not every client will start in the same phase of training, as many clients will already be regularly participating in cardiorespiratory exercise, and only clients with very specific performance-related objectives will reach the Performance Training phase.
When designing a Performance Training program for a client, the heart rates at VT1 and VT2 will be used as metabolic markers to differentiate between training zones 1, 2 and 3. For example, if a client’s heart rate at VT1 is 145 bpm and heart rate at VT2 is 164 bpm, the client’s heart rate zones would be as follows:
Zone 1: heart rate <145 bpm
Zone 2: heart rate = 145 to 163 bpm
Zone 3: heart rate ≥164 bpm
A client’s HR at VT1 and VT2 will increase as their fitness level improves, so it is important to periodically reassess those values as training progresses. In addition, for multisport athletes, conduct VT1 and VT2 assessments for each sport, as the heart rates will vary among training modalities.
For Performance Training, a good rule of thumb is to distribute the duration of training among the three zones according to the following format:
• Zone 1: 70–80% of training time
• Zone 2: <10% of training time
• Zone 3: 10–20% of training time
It might seem counterintuitive that a client will attain performance objectives when zone 1 training is 80% of the training time. By completing adequate zone 1 training, clients will have the mental and physical energy required to perform their zone 2 and 3 training bouts, which require high-intensity intervals that can place a large physical and mental toll on a client. This will also help to avoid overtraining.
Training in zone 1 should include warm-ups, cool-downs, long-distance workouts where the aim is endurance, and recovery bouts following the zone 2 and zone 3 interval bouts. The volume of training time is higher in zone 3 than zone 2 because work in zone 3 has been found to result in the greatest improvements in anaerobic capacity. The least amount of work is performed in zone 2 because evidence indicates these intensities elicit fewer training adaptations.
The frequency of zone 2 and 3 interval workouts is client-specific, based on the client’s goals, available training time, available recovery time and external factors. Zone 2 intervals are generally longer in duration, but lower in intensity than zone 3 intervals, while zone 3 intervals require longer recovery bouts. This article outlines a Performance Training program for a client interested in marathon training.
Exclusive for ACE Certified Professionals, the link at the top of the page features a case study example for a busy 39-year-old mother of three who wants to run a 5K race and how the ACE IFT Model can help move her from Base Training to Fitness Training to achieve her goal.
Final Thoughts
The evidence-based Cardiorespiratory Training component of the ACE IFT Model and its threshold-based programming approach makes it possible to design, implement and modify exercise programs based on the unique abilities, needs and goals of each client. Health and exercise professionals who align with the ACE Mover Method™ philosophy and embrace their skills in the ACE ABC Approach create client-centered interactions that establish rapport with their clients. Along with the ACE IFT Model Exercise Programming Template, you can design effective, individualized exercise programs that optimize your clients’ training responsiveness and achieve their health and fitness goals.
_____________________________________________________________
Gary P. Van Guilder, PhD, is an Associate Professor of Exercise and Sport Science at Western Colorado University. His research broadly focuses on developing better therapeutic strategies to improve vascular endothelial protection from ischemic injury. Therapeutic strategies span mechanical (remote ischemic preconditioning), pharmacological (lactate infusions), and lifestyle (diet and exercise) approaches.