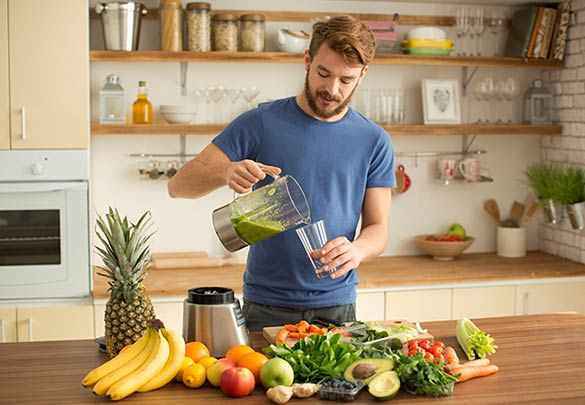
Exercise increases the concentration of molecules known as free radicals. A free radical is a molecule that’s missing at least one electron in its outermost orbit, which causes it to be negatively charged. Traditionally, research has linked increased production of free radicals with damage to DNA, increased muscle breakdown and accelerated aging. Accordingly, various strategies have been employed to not only reduce free radical production, but to also augment antioxidant concentrations. However, paradoxically, more recent research has demonstrated that increased free radical production post-exercise actually serves as an important signaling mechanism for favorable training adaptations. In other words, it might be time to reevaluate your assumptions about free radicals, antioxidants and exercise.
Free Radicals Explained
A free radical refers to atoms or groups of atoms that are highly reactive due to an unpaired valence electron (electrons in the outer shell of an atom that play key roles with interactions with other atoms or become paired within the same atom). Once formed, free radicals can create a domino effect of reactions, which may cause damage to biological cells. Within animal muscle tissue, there are five main radicals that that have a biological impact: superoxide, hydrogen peroxide, hydroxyl radical, nitric oxide and peroxynitrite (Gross, Baum and Hoppeler, 2011).
Exercise can lead to an increase in free radical production due to increased activation of aerobic bioenergetic pathways during endurance exercise, substrate depletion and exercise-induced hyperthermia. Additionally, transient hypoxia (low tissue oxygenation) during anaerobic exercise can lead to acidosis (Kanter, 1998), and the mechanical stress of exercise can increase oxidative stress and lead to free radical formation.
Due to their high reactivity, free radicals have associated negative biological effects. Free radicals are able to deform other biological molecules, causing the damage to the cellular structure and ultimately obstructing cellular function. For example, high oxidative stress and free radical production damages the unsaturated fatty acids located in the lipid bilayer of cell membranes, causing a leaky cellular structure (Valko et al., 2004). Furthermore, damage can be done with the oxidation of nucleotides, causing damage to DNA and resulting in the development of tumors (Valko et al., 2006). Free radicals can also be responsible for denaturing protein structure, which results in the proteins becoming non-functional. Ultimately, free radicals lead to cellular damage, which is a common pathway for cancer, aging and a variety of diseases.
Antioxidants Explained
Antioxidants are molecules that can scavenge and safely interact with free radicals. Specifically, antioxidants can help mitigate free radical-induced negative chain reactions and protect cell membranes from oxidative damage (Rodriguez, DiMarco and Langley, 2009). Antioxidants can be either endogenous (originating within the body) or exogenous (originating outside of the body, via nutritional habits or supplementation, for example). The main exogenous antioxidants include vitamins C and E, and beta-carotene (the precursor to vitamin A). Table 1 lists common food sources of these exogenous antioxidants.

Vitamin C may aid in strengthening the immune defense, and vitamin E may help with improving energy balance. Additionally, when consumed together, vitamins C and E can help protect against lipid peroxidation (i.e., leaky cell membranes) (Gross, Baum and Hoppeler, 2011). Chronic exercise training produces constant oxidative stress within the muscles and other cells due to a 10- to 15-fold increase in oxygen consumption associated with an exercise bout (Powers et al., 2004). In response to the constant oxidative stress produced during chronic exercise training, a well-trained individual will have a more highly developed endogenous antioxidant system to combat the free radical production compared to a sedentary individual (Rodriguez, DiMarco and Langley, 2009).
Commonly Held Beliefs About Free Radicals, Antioxidants and Exercise
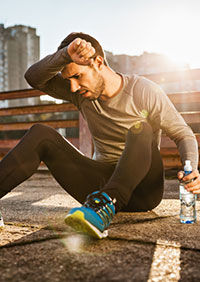
Historically, the common perception of free radicals and antioxidants and their interaction with exercise training has been a classic case of good guy vs. bad guy. For instance, in the scientific literature of the 1970s and 1980s, antioxidants were recognized as disease risk-lowering “allies,” while free radicals were largely considered disease-inducing “enemies” (Adams, Egbo and Demmig-Adams, 2014). The reasoning was that free radicals produced during and after strenuous exercise caused muscle damage and fatigue, which, in turn, led to decreased performance (Neubauer and Yfanti, 2015). Accordingly, it was surmised that supplementation with antioxidants could alleviate exercise-induced muscle damage, hasten training recovery and increase overall performance.
This original hypothesis, that antioxidant supplementation could combat exercise-related free-radical production while also augmenting exercise training and performance, prompted much research in the ensuing decades. Today, the scientific consensus is that antioxidant supplementation provides minimal or no beneficial effects. Nevertheless, the interest in antioxidant supplements among athletes and physically active individuals continues to be high. Indeed, the antioxidant supplement industry is now a multibillion dollar industry worldwide (Gross, Baum and Hoppeler, 2011). Moreover, it has been reported that antioxidants are among the most commonly used supplements among athletes (Braun et al., 2009).
Critically, it would appear that the lack of past scientific evidence supporting the practice of antioxidant supplementation, coupled with its paradoxical current widespread use, warrants a closer inspection of this issue. In the following sections, we’ll take a close look at more recent research examining the connections among free radicals, antioxidants and exercise training.
A Review of the Latest Research
Because of the long and well-established link between cell damage and free radicals, conventional wisdom has held that antioxidant supplementation may benefit exercise performance and enhance recovery by countering the increase in free radicals associated with exercise. As noted earlier, however, this premise has been unsupported by research. In fact, contemporary research is questioning the effectiveness of the antioxidant supplementation strategy altogether. In the last decade, for example, it has been demonstrated that consuming superfluous (i.e., excessive) doses of antioxidant supplements in an effort to retard free radical production during exercise training actually contributes to increased muscle fatigue and delayed recovery (Teixeira et al., 2009; Close et al., 2006). Moreover, it has also been reported that antioxidant supplementation with vitamin C hampers training-induced adaptations in endurance performance (Gomez-Cabrera et al., 2008).
These findings have led many experts to suggest that antioxidants might actually be detrimental to training and performance. Here are three more key studies that lend support to this view:
- A group of researchers in Spain performed a double-blind randomized control trial in which they examined the effectiveness of eight weeks of endurance training in a group that was supplemented with an oral dose of 1 g of vitamin C as compared to a group that was not supplemented. The group that was not supplemented exhibited an 11 percent greater improvement in maximal oxygen uptake (VO2max). The authors concluded that vitamin C supplementation decreases endurance-training efficiency (Gomez-Cabrera et al., 2008).
- English researchers investigated the effects of vitamin C supplementation on reactive oxygen species (ROS) production and delayed-onset muscle soreness (DOMS) following downhill running. Participants were assigned to one of two groups: (1) the ascorbic acid group (group AA), which received 1 g of ascorbic acid two hours before and for 14 days after downhill running; and (2) the placebo group (Pl group), which received a placebo. While both groups experienced DOMS and impaired muscle function post-exercise, the AA group also experienced delayed recovery. The authors concluded that vitamin C supplementation may hinder post-exercise recovery of muscle function (Close et al., 2006).
- A group of Portuguese researchers aimed to determine the effects of four weeks of antioxidant (AOX) supplementation on exercise-induced muscle damage and inflammation in kayakers. Participants were randomly assigned to receive a placebo or an AOX capsule. Before and after supplementation, blood samples were collected at rest and 15 minutes after a 1000-meter kayak race. It was found that AOX supplementation does not offer protection against exercise-induced inflammation and may impair the recovery of muscle damage (Teixeira et al., 2009).
Collectively, these findings strongly suggest that antioxidant supplementation hampers favorable exercise training adaptations and interferes with the recovery process. The next logical question is, of course, why?
Interestingly, as early as 1971, it was shown that antioxidant supplementation (400 IU/day of vitamin E supplementation for six weeks) caused unfavorable effects on endurance performance in swimmers (Sharman, Down and Sen, 1971). Overall, the authors concluded “there is no evidence here to suggest that vitamin E has any beneficial effect on endurance performance. Indeed the evidence, if anything, suggests that the vitamin has an unfavorable effect.”
Furthermore, over the past five to 10 years, numerous studies have shown that the exercise-induced increase in free radicals actually serves as an important signaling molecule that ultimately leads to favorable training adaptations (Powers et al., 2010; Ristow et al., 2009).
Could an increase in free radicals actually be a necessary evil required for favorable training adaptations? A growing body of evidence suggests that this may indeed be the case. For example:
- As mentioned in the previous section, Spanish researchers showed that improvements in VO2max were 11 percent lower following eight weeks of endurance training in a group that supplemented with antioxidants (vitamin C) when compared to a group that was not supplemented. Significantly, the authors identified that the underlying reason why vitamin C supplementation decreased endurance training efficiency was that it prevented crucial cellular adaptations to exercise (Gomez-Cabrera et al., 2008).
- German researchers evaluated the effects of a combination of antioxidants (vitamins C and E) on insulin sensitivity in both previously untrained and trained healthy young men, before and after a four-week exercise intervention. They found that exercise-induced ROS production improved insulin resistance and caused a beneficial adaptive response that promoted natural antioxidant defense capacity. They also noted that blocking exercise-dependent formation of ROS by ingesting antioxidant supplements abolished the health-promoting effects of exercise. Exercise increased the parameters of insulin sensitivity only in the absence of antioxidants in both previously untrained and trained participants. The authors concluded that exercise-induced oxidative stress helps to improve insulin resistance; however, antioxidant supplementation negates these health-promoting effects of exercise in humans (Ristow et al., 2009).
- American researchers investigated the effects of both antioxidant supplementation and chronic exercise training on blood pressure in a cohort of older, hypertensive men. Blood pressure was measured at rest and during exercise, with and without antioxidant supplementation (vitamins C and E, and α–lipoic acid). Following six weeks of exercise training, systolic and diastolic blood pressure each reduced by 12 mmHg. However, it was also reported that antioxidant supplementation administered following exercise training completely blunted these favorable training adaptations. The researchers concluded that an exogenous supply of antioxidants was counterproductive and interfered with the important signaling role that ROS (i.e., free radicals) played in arterial vasodilation (Wray et al., 2009).
Practical Applications for Health and Fitness Professionals
Given the compelling evidence produced over the past decade and presented here, we now understand that the relationships among free radicals, antioxidants and exercise are far more complex than once thought. It is not simply a case of good guy vs. bad guy (see Figure 1). Here are three important takeaway messages for health and fitness professionals to consider:
The dose of antioxidant matters. Similar to many other physiological phenomena, there appears to be a dose-response relationship at play between the consumption of antioxidants and the corresponding physiological response. Whereas a low dose of antioxidant has a beneficial effect, a high dose is detrimental (Neubauer and Yfanti, 2015). Indeed, in many of the studies where antioxidant supplementation was found to be harmful, the doses of antioxidants consumed were five to 17 times greater than the recommended dietary allowance (RDA). By contrast, it has been reported that doses of antioxidants at or near 100 percent of the RDA were sufficient for maintaining the body’s antioxidant defenses, even for competitive endurance athletes.
Clearly, given the potential harmful effects, the high doses of antioxidants frequently consumed by athletes and individuals participating in regular exercise should be avoided. In fact, in the words of expert researchers in this area, antioxidant supplementation is “worse than useless” (Gomez-Cabrera, Ristow and Viña, 2012).
The source of the antioxidant matters. Given that the majority of research over the past several decades has shown no beneficial effect of antioxidant supplementation, it has been recommended that the nutritive antioxidant requirements for individuals participating in exercise training should range between 100 to 200 percent of current recommendations for the general population. Moreover, research suggests that active individuals performing regular exercise training can maintain an appropriate physiological antioxidant status by eating a balanced and varied diet (Neubauer and Yfanti, 2015).
Acute and chronic elevations in oxidative stress elicit significantly different responses. It is well established that chronically elevated oxidative stress contributes to the pathophysiology of numerous diseases, including cancer, cardiovascular disease and diabetes. As such, it’s not altogether surprising that free radicals have gained such a widespread negative reputation. Nevertheless, it is exceptionally misleading to characterize any increase in free radical concentration as an adverse threat to other cells within the biological system. Critically, a number of recent studies have shown that an acute transient increase in ROS from exercise serves as an important signaling molecule, ultimately resulting in important physiological adaptations (Powers et al., 2010; Ristow et al., 2009).
Unfortunately, the long-held belief that all oxidative stress is harmful, and that preventing their actions is beneficial, has led to widespread consumption of antioxidants. Conversely, it appears that a transient, acute exercise-induced increase in free radicals is a positive and much needed metabolic event. It is long overdue that this one-sided thinking that free radicals only cause harm to the body should be dismissed.
Figure 1. An Overview of the Primary Signaling Pathways Related to Exercise, Oxidative Stress and Antioxidant Intake Via Nutritional Habits and Supplementation
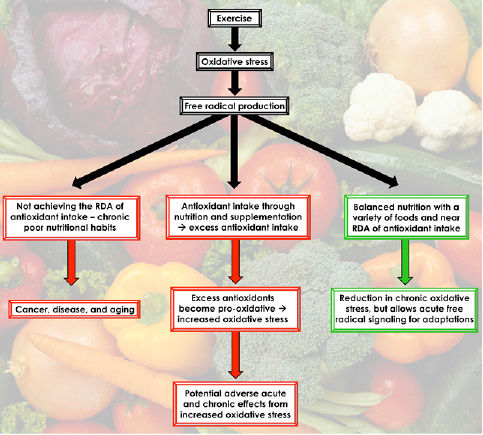
The pathways outlined in red could potentially stimulate adverse or harmful biological processes within the body. The pathway outlined in green, which features a balanced diet with a variety of foods, is recommended. The suggested recommended dietary allowance (RDA) of antioxidants is 75 to 90 mg of vitamin C, 15 mg of vitamin E and 700 to 900 µg of vitamin A (Fink and Mikesky, 2015).
References
Adams, R.B., Egbo, K.N. and Demmig-Adams, B. (2014). High-dose vitamin C supplements diminish the benefits of exercise in athletic training and disease prevention. Nutrition & Food Science, 44, 95-101.
Braun, H. et al. (2009). Dietary supplement use among elite young German athletes. International Journal of Sport Nutrition and Exercise Metabolism, 19, 97-109.
Close, G.L. et al. (2006). Ascorbic acid supplementation does not attenuate post-exercise muscle soreness following muscle-damaging exercise but may delay the recovery process. The British Journal of Nutrition, 95, 976-981.
Fink, H.F. and Mikesky, A.E. (2015). Practical Applications in Sport Nutrition (4th ed.). Burlington, Mass.: Jones & Bartlett Learning.
Gomez-Cabrera, M.C., Ristow, M. and Viña, J. (2012). Antioxidant supplements in exercise: Worse than useless? American Journal of Physiology. Endocrinology and Metabolism, 302, E476-477.
Gomez-Cabrera, M.C. et al. (2008). Oral administration of vitamin C decreases muscle mitochondrial biogenesis and hampers training-induced adaptations in endurance performance. The American Journal of Clinical Nutrition, 87, 142-149.
Gross, M., Baum, O. and Hoppeler, H. (2011). Antioxidant supplementation and endurance training: Win or loss? European Journal of Sport Science, 11, 27-32.
Jackson, M.J. (2009). Redox regulation of adaptive responses in skeletal muscle to contractile activity. Free Radical Biology & Medicine, 47, 1267-1275.
Kanter, M. (1998). Free radicals, exercise and antioxidant supplementation. The Proceedings of the Nutrition Society, 57, 9-13.
Neubauer, O. and Yfanti, C. (2015). Antioxidants in athlete’s basic nutrition: Considerations towards a guideline for the intake of vitamin C and vitamin E. In: Lamprecht, M. (Ed.). Antioxidants in Sport Nutrition, Chapter 3. Boca Raton, Fla.: CRC Press.
Powers, S.K. et al. (2010). Reactive oxygen species are signaling molecules for skeletal muscle adaptation. Experimental Physiology, 95, 1-9.
Powers, S.K. et al. (2004). Dietary antioxidants and exercise. Journal of Sports Sciences, 22, 81-94.
Ristow, M. et al. (2009). Antioxidants prevent health-promoting effects of physical exercise in humans. Proceedings of the National Academy of Sciences of the United States of America, 106, 8665-8670.
Rodriguez, N.R., DiMarco, N.M. and Langley, S. (2009). Nutrition and athletic performance. Medicine & Science in Sports & Exercise, 41, 709-731.
Sharman, I.M., Down, M.G. and Sen, R.N. (1971). The effects of vitamin E and training on physiological function and athletic performance in adolescent swimmers. The British Journal of Nutrition, 26, 265-276.
Teixeira, V.H. et al. (2009). Antioxidants do not prevent post-exercise peroxidation and may delay muscle recovery. Medicine & Science in Sports & Exercise, 41, 1752-1760.
Valko, M. et al. (2006). Free radicals, metals and antioxidants in oxidative stress-induced cancer. Chemico-Biological Interactions, 160, 1-40.
Valko, M. et al. (2004). Role of oxygen radicals in DNA damage and cancer incidence. Molecular and Cellular Biochemistry, 266, 37-56.
Wray, D.W. et al. (2009). Oral antioxidants and cardiovascular health in the exercise-trained and untrained elderly: A radically different outcome. Clinical Science (London, England: 1979), 116, 4.