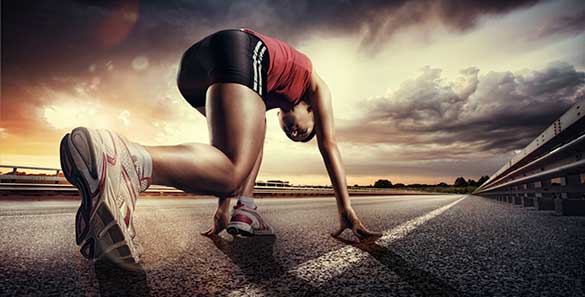
Endurance races have never been more popular, and chances are many of your clients have committed to running a half-marathon (or longer race) in the coming year. And they are looking to you for guidance on how to train for their chosen events. Performance in endurance-related activities is dependent on the triad of VO2max, lactate threshold and economy, and a growing body of research is helping pros fine-tune their clients’ training programs to maximize these factors. The purpose of this article is to provide you with the latest evidence-based training strategies for improving these key performance-defining physiological parameters.
The Physiology of Endurance Performance
Endurance exercise can be defined as the ability to perform any type of cardiovascular exercise (e.g., cross-country skiing, cycling, running, hiking, swimming) for an extended period of time (Robergs and Roberts, 1997). The complex integration of multiple physiological functions may limit an individual in the pursuit of these endurance exercises. Yet, despite its multifaceted nature, endurance performance is characterized by one simple requirement—the necessity to sustain repeated skeletal muscle contraction. Whether your client is an elite athlete, recreational runner or currently sedentary individual, the ability to maintain repeated skeletal muscle contraction, and thus his or her overall potential for performance in endurance exercise, can be explained by a similar set of physiological attributes: VO2max, lactate threshold and economy of movement. Ultimately, however, it is the overall capacity and interaction of each of these parameters that determines individual peak endurance performance. A brief primer on the physiology of VO2max, lactate threshold and economy is presented in the sidebar, "Endurance Exercise Performance."
A fundamental appreciation of these three critical performance-defining physiological parameters provides the requisite foundation for designing a comprehensive training program focused on optimizing endurance performance. The following section examines the scientific evidence illustrating the powerful relationships that exist among VO2max, lactate threshold and economy values and endurances performance.
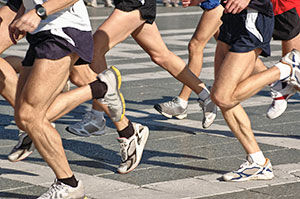
Endurance Exercise Performance
Maximal oxygen uptake (VO2max) refers to the highest rate at which oxygen can be taken up and consumed by the body during intense exercise (Bassett and Howley, 2000). Traditionally, the magnitude of an individual’s VO2max has been viewed as one of the most important predictors of endurance performance. Indeed, a classic study performed in the early 1970s at Ball State University confirmed the importance of VO2max to endurance performance, with findings indicating a strong correlation between VO2max and 10-mile run times (Costill, 1970).
The ability of the cardiorespiratory system to transport oxygen to the exercising muscles refers to the central component of VO2max (Robergs and Roberts, 1997). The role of the central component is for oxygen to be transported from the atmosphere and delivered to the muscles where it is utilized during mitochondrial respiration to produce adenosine triphosphate (ATP). The main central limitations to oxygen delivery are pulmonary diffusion, cardiac output, and blood volume and flow (Bassett and Howley, 2000). The ability of exercising muscles to extract and utilize oxygen, which has been transported by the cardiorespiratory system, refers to the peripheral component of VO2max (Robergs and Roberts, 1997). The potential sites for VO2max limitation in the peripheral component include muscle diffusion capacity, mitochondrial enzyme (molecules that facilitate ATP production in mitochondria) levels and capillary density (Bassett and Howley, 2000).
Lactate threshold refers to the intensity of exercise at which there is an abrupt increase in blood lactate levels (Robergs and Roberts, 1997). Many scientists consider the lactate threshold, relative to VO2max and economy, to be the strongest predictor of endurance performance (Kenney, Wilmore and Costill, 2015). Additionally, the lactate threshold (compared to VO2max and economy) appears to be the most responsive physiological parameter to endurance training (McArdle, Katch and Katch, 2014).
In untrained endurance individuals, the lactate threshold occurs at approximately 50 to 60 percent of VO2max. Following endurance training, individuals generally improve the lactate threshold to 75 percent of VO2max; with values at 80 to 90 percent of VO2max reported in elite, world-class endurance athletes (McKardle, Katch and Katch, 2014).
The premier performance benefit of this training adaptation is that an individual is capable of maintaining a higher steady-state exercise intensity (below the lactate threshold) during endurance exercise. This permits the endurance exerciser to maintain faster steady-state tempos during training or racing, leading to improved endurance performance. In fact, research has consistently reported high correlations between the lactate threshold and performance in a variety of endurance events including running, cycling and race-walking (McArdle, Katch and Katch, 2014). Furthermore, it has been proposed that the best predictor of endurance performance is the maximal steady-state workload achieved near VO2max (Weltman, 1995).
Economy is a term used to express the oxygen uptake required to perform a given exercise workload, whether it be cycling, running or another form of endurance activity (Daniels, 1985). Differences in oxygen consumption between individuals at similar exercise workloads illustrate the individual variation found in exercise economy. Consequently, individuals with similar VO2max values can have considerably different endurance performances depending on their economy of movement. In fact, high correlations between 10K running performance and economy have been reported between runners with comparable VO2max values (Conley and Krahenbuhl, 1980). Individual exercise economy is enhanced with endurance training and has been explained by improvements in biomechanical techniques in performing the specific physical activity (Robergs and Roberts, 1997).
Why Does Increased VO2max-lactate Threshold Economy Equate to Improved Performance?
There is a strong relationship between aerobic performance and VO2max (Costill, 1970), lactate threshold (Kenney, Wilmore and Costill, 2015) and economy (Bassett and Howley, 2000). Furthermore, there is a direct relationship between running economy based on VO2max and lactate threshold values. VO2max sets the upper limit for endurance performance and it has been found for every one-point increase in VO2max, individuals took 10 seconds off a 10-mile run (Costill, 1970).
Lactate threshold represents the percent VO2max at which an abrupt increase in blood lactate levels is seen. There is overwhelming evidence linking lactate threshold with endurance performance, particularly for running events (Faude, Kindermann and Meyer, 2009). Economy could be noted as the most important of the aerobic training triad, as there is a direct relationship between increases or decreases in VO2max and lactate threshold, as well as biomechanical techniques in performance (Robergs and Roberts, 1997).
While it has been well established that VO2max alone has a strong relationship with endurance performance, it is important to understand the interplay between the VO2max, lactate threshold and economy triad and overall endurance performance. For example, Participants A and B both have a VO2max of 45 mL/kg/min. In theory, they should perform relatively similarly in an endurance event (e.g., a half-marathon). However, Participant A has a lactate threshold that occurs at 50 percent of VO2max, while Participant B’s lactate threshold occurs at 75 percent of VO2max. In this situation, near their lactate thresholds, Participant A would have a velocity of 3.9 mph (104.5 m/min), while Participant B would have a velocity of 5.8 mph (155.4 m/min). Therefore, even though the VO2max values are the same for both participants, there is a large variability in performance due to Participant B’s ability to maintain a running velocity that is nearly 2 mph faster (53.6 m/min). Consequently, it is not only important to have a high aerobic capacity, but also to be able to sustain a high relative maximal capacity (lactate threshold at a high percent VO2max) to better enhance economy and performance. Understanding the mechanisms for improving the VO2max, lactate threshold and economy triad and recognizing the interplay between the triad and overall endurance performance is critical to designing an evidence-based training protocol.
Top Training Strategies to Increase VO2max
Traditionally, a primary exercise training strategy for improving VO2max has been to increase overall training volume (increased frequency and longer duration) (Garber et al., 2011). This approach has been applied to both the general and athletic populations. For instance, Dalleck and colleagues (2009) demonstrated that a greater increase in training volume (45 minutes, five days/week compared to 30 minutes, five days/week) improved VO2max by nearly twice as much (23.4 percent vs. 12.6 percent) in previously sedentary women. Likewise, it has been shown that there was a 5 percent continued improvement in VO2max in Olympic-caliber endurance athletes after completing eight weeks of high volume (~110 miles/week) training (Billat et al., 2002).
However, contemporary research has been focusing on the implementation of low-volume, high-intensity training to improve VO2max. In fact, it has been shown that high-intensity intervals [47 x 15-second intervals or 4 x 4-minute intervals at 90 to 95 percent heart-rate max (HRmax)] are significantly more effective at improving VO2max compared to long, slow distance running (45 minutes at 70% HRmax). These results have been consistent among a variety of studies investigating high-intensity interval training, with proposed improvements in VO2max linked to increases in cardiac output (amount of blood pumped by the heart per minute) and increased muscle oxidative potential (ability of active tissue to extract oxygen from the blood) (Sloth et al., 2013). Could low-volume, high-intensity interval training be more effective at improving VO2max than traditional exercise-training strategies? Here are two key studies that address this question:
- A group of researchers (Helgerud et al., 2007) investigated the effects of high-intensity interval training compared to traditional aerobic training. Participants completed three training sessions per week for eight weeks and were assigned to one of four groups: (1) long slow distance of 45 minutes at 70 percent HRmax, (2) lactate threshold running at 85 percent HRmax for 24.25 minutes, (3) 47 repetitions of 15-second intervals at 90 to 95 percent HRmax with 15 seconds of active recovery, and (4) 4 x 4-minute interval training at 90 to 95 percent HRmax with three minutes of active recovery. It was found that both the 47 x 15-second and 4 x 4-minute high-intensity groups had a significant increase in absolute VO2max (5.5 percent and 7.3 percent, respectively) compared to moderate- and low-intensity training over an eight-week period.
- Canadian researchers (Hazell et al., 2010) investigated three types of sprint-interval (high-intensity) training. Participants completed three training sessions per week for two weeks in one of the following experimental groups, with workload set at 100 g/kg of body mass cycling: (1) 30 seconds all-out with four minutes of active recovery, (2) 10 seconds all-out with four minutes of active recovery, and (3) 10 seconds all-out with two minutes of active recovery. Progression was completed by increasing the number of repeat sprints from four to six repetitions for the final two training sessions. They found that the two 10-second intervention groups had similar increases in VO2max (4 to 9 percent) when compared to the standard 30-second protocol. The results of this study indicate that VO2max can be improved with only two weeks of sprint-interval training.
Strategies for Increasing Lactate Threshold
Training elicits improvements in the lactate threshold through a number of mechanisms, including greater mitochondrial density, increased ratio of type I to type II muscle fibers and enhanced capillary density surrounding the type I muscle fibers (Ivy et al., 1980). Traditionally, the key to optimizing lactate threshold has been base training (consisting of high-volume, moderate-intensity exercise), steady-state lactate threshold sessions and interval workouts at an intensity above the lactate threshold (Dalleck and Kravitz, 2009). While this paradigm has not been overturned, here are two key studies that expand our lactate threshold training knowledge and focus on the increasingly popular high-intensity, low-volume training protocols:
- Researchers in New Zealand (Barnes et al., 2013) compared the effectiveness of various uphill interval-training protocols on numerous markers of performance, including velocity at lactate threshold. The participants, all young athletic males, were split into five groups and performed two weekly sessions of uphill intervals for six weeks. The uphill interval-training protocols are presented in Table 1, including the weekly progressions of interval-training parameters for each group. At the conclusion of the intervention, the velocity at lactate threshold was most improved in groups 3 and 4 (highlighted in table). Moreover, the improvement in velocity at lactate threshold corresponded to a superior endurance running performance, as evidenced by an approximately 20-second faster 5K time trial. In summary, it was recommended that an optimal protocol for uphill interval training to improve velocity at lactate threshold entails four to nine interval bouts of two to five minutes, each performed at an intensity corresponding to 93 to 100 percent HRmax (e.g., 7 to 10 percent treadmill grade).
- German researchers (Hottenrott et al., 2012) compared the improvements in velocity at lactate threshold in a cohort of recreationally active subjects performing short, intensive training sessions vs. continuous endurance running. Both groups performed equal volumes of training, at 2.5 total hours weekly. One group performed five short, high-intensity sessions (e.g., 4 to 6 x 2-minute intervals at 100 percent HRmax interspersed with 90 seconds of running at 85 percent velocity at lactate threshold) during the week, while the other performed two longer endurance-training sessions on the weekend. The velocity at lactate threshold improved more significantly in the intensive training group (20.6 percent increase) when compared to the continuous endurance running group (13.1 percent increase). It was concluded that the nearly twofold greater increase in the intensive-training group confirms the importance of training both above and at the lactate threshold to elicit positive improvements in this critical physiological marker.
Strategies for Improving Economy
While improvements to VO2max and lactate threshold represent better efficiency at regenerating ATP for muscular contraction, improving economy benefits performance by reducing the energy necessary to sustain the same workload or velocity. Economy is impacted by adaptations of the metabolic, cardiorespiratory, biomechanical and neuromuscular systems. Adaptations to these systems can be achieved through a variety of training interventions, including endurance training, resistance training and high-intensity interval training, and are related to exposure of differing environmental conditions (e.g., altitude, heat, mixed terrain). Additionally, flexibility and some nutritional interventions (caffeine and dietary nitrate intake) can also impact economy (Barnes and Kilding, 2015). Recently, there has been increased interest in the impact of resistance training, high-intensity interval training and stretching on neuromuscular recruitment and the effects on endurance performance. Here are two key articles:
- Brazilian researchers (Damasceno et al., 2015) evaluated the impact of an eight-week strength-training intervention on the neuromuscular aspects of pacing and running economy in a 10K time trial. Both the strength-training group (STG) and control group were instructed to maintain their current endurance aerobic-training schedules, while the STG performed two additional weekly strength-training sessions consisting of half-squat, leg press, plantar flexion, and knee extension exercises. The load and repetitions progressed every two weeks with the following sequence: three sets of eight to10 repetitions (3 x 8-10), 3 x 6-8, 3 x 4-6 and 2 x 3-5. Following the intervention, there was an improvement of 2.5 percent and a decrease of 0.7 percent in the 10K time-trial performance for the STG and control group, respectively. It was concluded that all of the improvement in the 10K time trial performance in the STG group resulted from an ability to sustain a faster pace during the last third of the time trial and could be directly attributable to increased running economy.
- A recent review (Carter and Greenwood, 2015) examined the effects of multiple forms of stretching (static and dynamic) and flexibility on running economy. It was found that there is plentiful evidence to suggest that static stretching prior to exercise can significantly decrease economy by reducing elastic energy return. However, overall flexibility can benefit economy by increasing range of motion at the higher velocities associated with race pace. Additionally, a dynamic warm-up and dynamic stretching prior to performance may increase economy by optimizing recruitment patterns in the muscles targeted.
Conclusion
VO2max, lactate threshold and economy are the most important determinants of success in endurance-related activities and events. Not only are these physiological parameters individually important, the interaction between the triad also plays a critical role in endurance performance. By understanding the mechanisms of these physiological attributes, combined with the latest research on how these parameters can be improved, you will be better equipped to design effective and comprehensive training programs for your endurance-minded clients.
References
Barnes, K.R. et al. (2013). Effects of different uphill interval-training protocols on running economy and performance. International Journal of Sports Physiology and Performance, 8, 639-647.
Barnes, K.R. and Kilding, A.K. (2015). Strategies to improve running economy. Sports Medicine, 45, 37-56.
Bassett Jr., D.R. and Howley, E.T. (2000). Limiting factors for maximum oxygen uptake and determinants of endurance performance. Medicine & Science in Sport & Exercise, 32, 70-84.
Billat, V. et al. (2002). Effect of training on the physiological factors of performance in elite marathon runners (males and females). International Journal of Sports Medicine, 23, 336-341.
Carter, J. and Greenwood, M. (2015). Does flexibility exercise affect running economy? A brief review. Strength and Conditioning Journal, 37, 12-21.
Conley, D.L. and Krahenbuhl, G. (1980) Running economy and distance running performance of highly trained athletes. Medicine & Science in Sport & Exercise, 12, 357-360.
Costill, D.L. (1970). Metabolic responses during distance running. Journal of Applied Physiology, 28, 251-255.
Dalleck, L.C. et al. (2009). Dose-response relationship between moderate-intensity exercise duration and coronary heart disease risk factors in postmenopausal women. Journal of Women’s Health, 18, 105-113.
Dalleck, L.C. and Kravitz, L. (2009). How to design a lactate-threshold training program. ACE Certified News, 3-6, 24.
Damasceno, M.V. et al. (2015). Effects of resistance training on neuromuscular characteristics and pacing during 10-km running time trial. European Journal of Applied Physiology, 115, 1513-1522.
Daniels, J. (1985). A physiologist’s view of running economy. Medicine & Science in Sport & Exercise, 17, 332-338.
Faude, O., Kindermann, W. and Meyer, T. (2009). Lactate threshold concepts: How valid are they? Sports Medicine, 39, 469-490.
Garber, C.E. et al. (2011). Quantity and quality of exercise for developing and maintaining cardiorespiratory, musculoskeletal and neuromotor fitness in apparently healthy adults: Guidance for prescribing exercise. Medicine & Science in Sport & Exercise, 47, 1334-1359.
Hazell, T.J. et al. (2010). 10 of 30-s sprint interval training bouts enhance both aerobic and anaerobic performance. European Journal of Applied Physiology, 110, 153-160.
Helgerud, J. et al. (2007). Aerobic high-intensity intervals improve VO2max more than moderate training. Medicine & Science in Sports & Exercise, 39, 665-671.
Hottenrott, K., Ludyga, S. and Schulze, S. (2012). Effects of high-intensity training and continuous endurance training on aerobic capacity and body composition in recreationally active runners. Journal of Sports Science and Medicine, 11, 483-488.
Ivy, J.L. et al. (1980). Muscle respiratory capacity and fiber type as determinants of the lactate threshold. Journal of Applied Physiology: Respiratory, Environmental and Exercise Physiology, 48, 523-527.
McArdle, W.D., Katch, F.I. and Katch, V.L. (2014). Exercise Physiology: Energy, Nutrition and Human Performance. Baltimore, Maryland: Williams and Wilkins.
Robergs, R.A. and Roberts, S. (1997). Exercise Physiology: Exercise, Performance and Clinical Applications. St Louis, Mo.: Mosby.
Sloth, M. et al. (2013). Effects of sprint interval training on VO2max and aerobic exercise performance: A systemic review and meta-analysis. Scandinavian Journal of Medicine and Science in Sports, 23, e341-e352.
Weltman, A. (1995). The Blood Lactate Response to Exercise. Champaign, Ill.: Human Kinetics.
Kenney, W.L., Wilmore, J.H. and Costill, D.L. (2015). Physiology of Sport and Exercise. Champaign, Ill.: Human Kinetics.