
By Lance C. Dalleck, Ph.D.
Simply defined, yet physiologically quite complex, fatigue refers to the inability to continue exercise at a given intensity. In all sports and exercise training, the onset of fatigue will vary depending on a person’s fitness level, the exercise intensity and duration, and environmental conditions (e.g., heat and humidity). This article provides a brief overview of the various types of fatigue and examines the role gender plays in fatigue. Also covered are training recommendations for combatting fatigue. (Note: It might be helpful to briefly review the primer on the three energy pathways featured in Training Recovery: The Most Important Component of Your Clients’ Exercise Programs, which was published in the August issue of ACE Certified News.)
Three Primary Exercise Scenarios Associated With Fatigue
Fatigue is a process that develops over time, and is largely dependent on the duration and intensity of exercise. The three dominant exercise scenarios leading to fatigue are short-term intense exercise, repeated-sprint exercise and extended submaximal exercise (Girard et al., 2011; Robergs and Roberts, 1997). Following is an overview of the key metabolic and system events that contribute to fatigue under these circumstances.
Short-term Intense Exercise
During activities such as sprinting and high-intensity resistance exercise, continued muscle contraction is dependent on the formation of adenosine triphosphate (ATP) for the demanding energy needs. Under these exercise conditions, creatine phosphate (CrP), which resynthesizes ATP, and glucose breakdown (called glycolysis) are primarily responsible for maintaining ATP levels. Skeletal muscle concentrations of CrP are quite limited; in fact, resting CrP stores can be depleted within approximately 10 seconds (Powers and Howley, 2012). To offset a potential ATP deficiency, glycolytic flux rapidly increases, which subsequently results in the accumulation of lactate and hydrogen proton (H+) molecules. Historically, it was thought that lactate was principally a dead-end waste product that caused muscle soreness and fatigue. However, more contemporary research has shown lactate production to be beneficial to the performance of continued high-intensity exercise (see sidebar, “Does Lactate Cause Fatigue?”). Conversely, proton accumulation contributes to a decreased cellular pH (acidosis), which interferes with normal skeletal muscle contraction through a number of mechanisms:
1. Activity of the enzyme myosin ATPase is impaired. This enzyme is responsible for the breakdown of ATP in skeletal muscle and the release of energy to support muscle contraction.
2. Other enzymes involved in the cellular regulation of sodium and potassium during muscle contraction become impaired.
3. The protons produced from the increased glycolysis often interfere with calcium ions reacting with proteins at the myofilament site in muscle where contraction is occurring, further inhibiting the muscle’s ability to contract.
Training can partially mitigate these negative events. Check out the box below for advice on how to combat fatigue during short-term, intense exercise.
Fatigue-fighting Training Tip #1
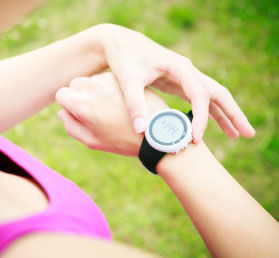
Improvements to the buffering-capacity system of the cellular environment will permit better tolerance of increased acidosis and, as a result, delay fatigue when performing short-term intense exercise. Interestingly, past research has shown that eliciting acidosis (i.e., high concentrations of protons) is a requisite training stimulus. To elicit favorable muscle buffering−capacity adaptations, have clients perform the following type of interval-training workout: Six to 10 bouts of two minutes each at a workload corresponding to 90 to 95% maximal heart rate with one minute rest. It is important to note that intensities below 80 to 85% or near or at 100% maximal heart rate will be inadequate for beneficial training adaptations to occur (Edge et al., 2006).
Repeated-sprint Exercise
Repeated-sprint exercise (RSE) is a fitness attribute required for success in many team and racquet sports (Girard et al., 2011). RSE is characterized by numerous short-duration sprints (generally lasting less than 10 to 15 seconds) interspersed with brief and incomplete recovery (typically less than 60 seconds). Many of the sources of fatigue for RSE are similar to those encountered in short-term intense exercise, including proton accumulation and a finite CrP supply. Exercise conditions, such as RSE, that extensively tax the phosphagen system ultimately lead to depletion in the primary fuel (i.e., CrP) for this energy pathway. Therefore, recovery from CrP degradation becomes critical if the later bouts of RSE are to be maintained at a high performance. Fatigue-fighting training tip #2 (below) highlights how combatting fatigue during RSE can be augmented.
A neural fatigue also exists during RSE. Each single motor nerve (which are under your voluntary control) activates a group of muscle fibers (ranging from a few to several hundred or more), and is collectively referred to as a motor unit. The chemical messengers, or neurotransmitters, that carry the motor nerve’s excitation message to the muscle at the neuromuscular junction, also can become impaired with RSE. This inhibition results in a decreased efficiency of the muscle fibers’ ability to contract during the later bouts of RSE.
Fatigue-fighting Training Tip #2
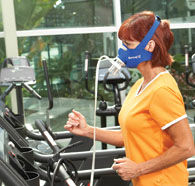
Research suggests that individuals with higher maximal oxygen uptake (VO2max) values have a greater capacity to resist fatigue during RSE. The most likely reason is that those with high VO2max levels also more rapidly resynthesize CrP stores between brief bouts of intense exercise. Traditionally, increasing cardiorespiratory fitness has been accomplished through the performance of moderate-intensity continuous exercise training. More contemporary research has shown interval training to also be a great option for quickly improving VO2max. The following workout has been shown to be particularly effective: Three to five 5-minute interval bouts at 90 to 95% maximal heart rate, with five minutes of active recovery at an intensity corresponding to 60 to 70% maximal heart rate. This workout should be performed one to two days per week (Gormley et al., 2008).
Extended, Submaximal Exercise
During prolonged exercises such as cycling, cross-country skiing and distance running, muscle contraction is also dependent on the ability of metabolic pathways (breakdown of a fuel to release energy) to continuously regenerate ATP. Mitochondrial respiration (aerobic metabolism in the mitochondrion of the cell) becomes the primary supplier of ATP. Many fuels, or substrates from fat, carbohydrates and proteins, are available for mitochondrial respiration; however, the two most important relative to fatigue are blood glucose and muscle glycogen. Fats in the form of triglycerides are also readily available for ATP production, but their breakdown is much slower than glucose and glycogen. Decreased levels of blood glucose and low levels of muscle glycogen have been more associated with the onset of fatigue in sustained exercise events.
In terms of optimizing exercise performance, a limiting factor to glycolysis, and maintenance of this maximal rate of ATP regeneration, is the substrate availability of muscle glycogen. Research has reported that muscle glycogen stores become depleted after approximately two hours of maximal usage (Fitts, 1994). Prolonged aerobic and resistance exercise (eccentric muscle contractions, in particular) can lead to substantial disturbances in the skeletal muscle environment, including damage to the sarcolemma, contractile proteins and connective tissue (Powers and Howley, 2012). These disturbances can impair the ability to transport blood glucose into the skeletal muscle cell, which in turn leads to a decreased capacity to replenish glycogen stores. As a result, fatigue may continue for numerous days after the workout.
Some exercise activities, such as hiking, cross-training events and endurance races, may take place in hot, humid environments that can lead to considerable heat stress and possibly dehydration. Insufficient fluid intake and replacement coupled with increased sweat rates will impair bodily temperature−control systems and cardiovascular function. Repeated muscle contractions during prolonged exercise leads to continuous, metabolically generated heat, which can dramatically increase core body temperature (a condition known as hyperthermia). Research suggests that rising core temperatures may cause fatigue in both the contracting muscles and central nervous system (Powers and Howley, 2012). Failure to maintain fluid balance throughout prolonged sports events and exercise sessions may eventually result in decreased availability of blood flow to both exercising muscles and the skin for dissipation of heat. This dehydration may also lead to higher heart rates and stress on the cardiovascular system (due to the lack of blood volume since blood is approximately 50% water) as the event continues. The importance of fluid intake for total-body health, sport performance and reduced fatigue cannot be overemphasized. The third training tip, below, explains how to improve exercise tolerance during exposure to hot and humid environments.
Fatigue-fighting Training Tip #3
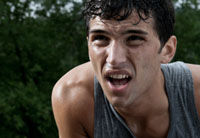
Chronic adaptations associated with heat acclimation include increased plasma volume, earlier onset of sweating during exercise combined with enhanced sweat rate, and decreased electrolyte loss. The stimulus required for eliciting these favorable adaptations is training in hot environments. The scientific literature has clearly shown, however, that the intensity of these training sessions need not be high. For example, it was recently reported (Lorenzo et al., 2010) that 10 days of moderate-intensity exercise training (~60 to 70% maximal heart rate) in a hot environment increased time-trial performance and VO2max (under hot environmental conditions). These findings are comparable to previous research. If you work with clients who will be competing in events that are likely to take place in hot environments, train them in similar conditions beforehand. It is recommended that moderate-intensity continuous exercise be performed at 60 to 70% maximal heart rate for 30 to 60 minutes per day (depending on the fitness level of your client) for at least one week in advance, but two weeks is ideal.
Armed with knowledge of the primary sources of fatigue during the most common exercise scenarios, it is important to examine some of the key gender differences related to fatigue that may exist among your clients.
Fatigue-related Differences (and Similarities) Between Men and Women
There are notable physiological differences between men and women in terms of muscle performance. Generally, men produce greater absolute muscle strength and power outputs relative to their counterparts (Billaut and Bishop, 2009). Conversely, women exhibit greater resistance to skeletal muscle fatigue and also demonstrate a superior capacity for recovery from exercise (Hunter and Enoka, 2001). Much of these contrasting properties can be explained by differences in muscle fiber types, and the corresponding energy system profile, that exist between men and women.
Does Lactate Cause Fatigue?
The classical explanation for the cause of fatigue, marked by sensations of pain and the muscle ‘burn’ experienced during intense exercise, was lactic acid build-up. Many coaches, athletes, exercise professionals and scientists alike have traditionally linked lactic acidosis with an inability to continue exercise at a given intensity. Although the lactate threshold indicates that conditions within the muscle cell have shifted to a state favorable for the development of acidosis, lactate production itself does not directly contribute to the fatigue experienced at high intensities of exercise. Interestingly, the molecule lactic acid is not actually even produced in the human body. At the normal range of muscle pH, it is the molecule lactate that is formed. Therefore, it is not sound science to use the terms lactic acid and lactate interchangeably.
Briefly, skeletal muscle can be divided into two different fiber types: type I (also commonly referred to as slow-twitch) and type II (also commonly referred to as fast-twitch). The type II fiber type can be further subdivided into type IIA and type IIX. The biochemical and enzymatic profile of each fiber type differs considerably. Type I muscle fibers are more suited to the catabolism of lipid and carbohydrate for ATP resynthesis through mitochondrial respiration. On the other hand, type II muscle fibers are better designed for regenerating ATP via the phosphagen system and glycolysis. Previous research has shown a greater distribution of type I muscle fibers and lower distribution of type IIX muscle fibers in women compared to men (Hick et al., 2001). Given these differences, it might seem intuitive that men have a greater potential for sprint- and strength-related performance, while women are better geared for endurance performance. Indeed, when performing the same relative muscular work, females tend to produce lower absolute muscle forces compared to males. This results in less oxygen demand in the exercising muscles. During submaximal exercise, these conditions enhance both oxygen delivery and metabolic by-product removal (primarily carbon dioxide), resulting in the delayed onset of fatigue. This apparently describes a female ‘fatigue resistance’ advantage in submaximal sporting and exercise events.
Additional gender differences tend to predispose females to success in extended submaximal activities (e.g., long-distance swimming and ultra-marathon running). Key enzymes involved in glycolysis, including phosphorylase and phosphofructokinase, have been found to be higher in men compared to women (Jaworowski et al., 2002). Further, lactate dehydrogenase activity has also been found to be greater. Collectively, these three key enzymatic differences contribute to an overall greater potential to regenerate ATP via glycolysis. Alternatively, no differences have been reported in the key enzymes involved in mitochondrial respiration, including those found in ß-oxidation, the Krebs cycle and the electron transport chain (Carter et al., 2001). Because of a greater distribution of type I fibers and lower type IIX fibers, along with the previously discussed sex differences in enzymatic activity, women are more dependent on mitochondrial respiration for ATP resynthesis. And although the maximal rate of ATP regeneration from mitochondrial respiration is slower compared to glycolysis, lesser reliance on this pathway means sparing of muscle glycogen and a delay in fatigue. Following both maximal and submaximal exercise, studies have reported that males tend to become more fatigued than females with regards to neuromuscular activation (Hick et al., 2001).
Lastly, endocrine status is another obvious difference between genders. For example, estrogen increases the concentrations of growth hormone, which concomitantly stimulates fat breakdown while reducing muscle glycogen breakdown. At first glance, this would be another reason why women are predisposed to better endurance performance. However, research has shown that differences in growth hormone release exist only at rest; with the onset of exercise, growth hormone concentrations are comparable between sexes. Finally, research remains equivocal with regards to the effects of different phases of the menstrual cycle on performance and fatigue (Billaut and Bishop, 2009).
References
Billaut, F. and Bishop, D. (2009). Muscle fatigue in males and females during multiple-sprint exercise. Sports Medicine, 39, 4, 257−278.
Carter, S.L. et al. (2001). Changes in skeletal muscle in males and females following endurance training. Canadian Journal of Physiology and Pharmacology, 79, 5, 386−392.
Edge, J., Bishop, D. and Goodman, C. (2006). The effects of training intensity on muscle buffer capacity in females. European Journal of Applied Physiology, 96, 97−105.
Fitts, R.H. (1994). Cellular mechanisms of fatigue. Physiology Review, 74, 1, 49−94.
Girard, O., Mendez-Villanueva, A. and Bishop, D. (2011). Repeated-sprint ability–part I: Factors contributing to fatigue. Sports Medicine, 41, 8, 673−694.
Gormley, S.E. et al. (2008). Effect of intensity of aerobic training on VO2max. Medicine and Science in Sports and Exercise, 40, 7, 1336–1343.
Hicks, A.L., Kent-Braun, J. and Ditor, D.S. (2001). Sex differences in human skeletal muscle fatigue. Exercise and Sports Sciences Review, 29, 3, 109−112.
Hunter, S.K. and Enoka, R.M. (2001). Sex differences in the fatigability of arm muscles depends on absolute force during isometric contractions. Journal of Applied Physiology, 91, 6, 2686−2694.
Jaworowski, A. et al. (2002). Enzyme activities in the tibialis anterior muscle of young moderately active men and women: Relationship with body composition, muscle cross-sectional area and fibre type composition. Acta Physiologica Scandinavica, 176, 215−225.
Lorenzo, S. et al. (2010). Heat acclimation improves exercise performance. Journal of Applied Physiology, 109, 1140−1147.
Powers, S.K. and Howley, E.T. (2012). Exercise Physiology: Theory and Application to Fitness and Performance (8th ed.). New York: McGraw-Hill Companies.
Robergs, R.A. and Roberts, S. (1997). Exercise Physiology: Exercise, Performance, and Clinical Applications. St. Louis, Mo.: Mosby.
____________________________________________________________________

Lance C. Dalleck, Ph.D., ACSM-RCEP, is academic coordinator of the Cardiac Rehabilitation postgraduate program at the University of Auckland in New Zealand. His research interests include improving exercise performance and health outcomes through evidence-based practice, quantifying the energy expenditure of outdoor and non-traditional types of physical activity, and studying historical perspectives in health, fitness and exercise physiology.