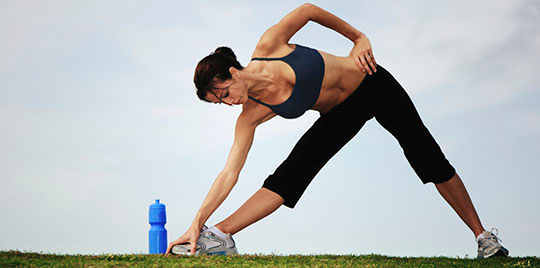
By Lance C. Dalleck, Ph.D. and Jeffrey M. Janot, Ph.D.
JOIN THE CONVERSATION!
What myth or misconception about health and fitness would you like to see banished for good? Share your thoughts in the comments section below.
A myth can be defined as an untrue explanation for a natural phenomenon. Unfortunately numerous myths remain pervasive and deeply engrained in the health and fitness world. In this first of a two-part series, we take a hard look at four longstanding myths to determine if they can withstand the weight of scientific scrutiny.
Myth #1: Stretching before exercise reduces the risk of injury.
Historically, it has been generally accepted that stretching decreases the risk of injury. Accordingly, it is common practice for stretching exercises to be included in a warm-up session. In fact, it has been suggested that stretching is the most common routine recommended by sports coaches and sports medicine professionals (Witvrouw et al., 2004). This myth is based on the idea that pre-exercise stretching reduces the risk of injury through improvements in range of motion and blood flow, better proprioception and decreased stiffness in the muscle (Fredette, 2001).
The fact that authority figures (e.g., coaches and sports medicine doctors) have long advocated pre-exercise stretching for lowering injury risk is likely the reason it has been so widely accepted as standard practice. In the late 1990s and early 2000s, however, researchers began to more closely scrutinize the evidence supporting this practice. For example, a review of existing literature led one group of researchers to conclude that pre-exercise static stretching does not lower the risk of local muscle injury (Shrier, 1999). Similarly, another group of scientists (Pope et al., 2000) reported no significant reductions in the incidence of lower-limb injuries in people who stretched before exercise compared to those who performed no stretching. More recently, Witvrouw and colleagues (2004) concluded that pre-exercise stretching has no beneficial effect on injury prevention for activities such as cycling, jogging and swimming. Finally, in a current review (McHugh and Cosgrave, 2010), the general consensus was that stretching in addition to aerobic warm-up does not affect the incidence of overuse injuries.
Clearly, the scientific literature of the past decade fails to support stretching before exercise as a successful strategy for injury prevention. Does this mean stretching has no impact on risk of injury? Absolutely not! In fact, it has been reported that stretching at other times, including postexercise and in the evening, can reduce injury risk.
The Bottom Line: Stretching before exercise DOES NOT reduce the risk of injury
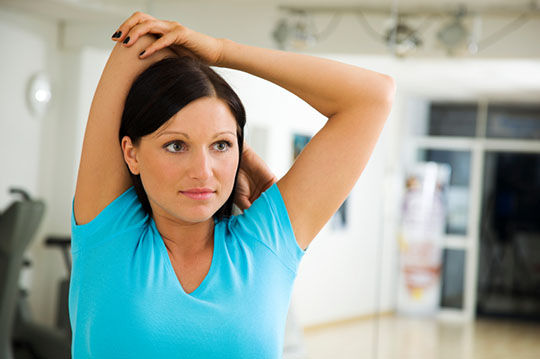
Myth #2: Static stretching improves exercise performance.
In addition to reducing the risk of injury, static stretching, as part of a pre-exercise warm-up routine, has also been recommended for improving exercise performance (American College of Sports Medicine, 2010; Shrier, 2004). Similar to myth #1, this myth involves claims that pre-exercise stretching enhances performance through improvements in range of motion and blood flow, better proprioception and decreased stiffness in the muscle (Fredette, 2001). For some, a feeling of “looseness” in the muscle prior to exercise equals better performance.
However, pre-exercise static stretching has actually been shown to negatively affect both explosive activities (jumping or sprinting) and maximal strength production (Shrier, 2004). Less is known about the effects of static stretching on endurance performance or balance. In addition, recent research suggests that the duration of hold while stretching is an important factor in determining the magnitude of effect on exercise performance (Kay and Blazevitch, 2012).
Static stretching affects muscular function in two separate ways: (1) decreased muscle stiffness (mechanical factor) and (2) decreased neuromuscular activation (neural factor) (Marek et al., 2005; Fletcher and Jones, 2004; Shrier, 2004).
Mechanical factors: The first post-stretching mechanism that elicits changes in exercise performance is the transient loss of stiffness in the musculotendinous unit (Little and Williams, 2006; Marek et al., 2005; Shrier, 2004). Decreased muscular stiffness increases the length of individual sarcomeres, which lowers actin and myosin cross-bridging, and negatively impacts the length-tension relationship within muscle. For explosive activities such as jumping or sprinting, stretching can inhibit the ability of the muscle to fully shorten in rapid fashion due to the lengthening of the muscle fiber induced by stretching. Additionally, the stretch-shortening cycle is influenced because decreased stiffness causes a decline in eccentric force development. The overall outcome of these mechanical events is decreased force and power production (Shrier, 2004).
Neural factors: The second mechanism induced by static stretching is decreased neural activation of muscle. The primary theory states that stretching elicits a neuroinhibitory effect that can be measured by decreases in neural signaling to the muscle. Neural activation is measured with a tool called electromyography (EMG), which involves the placement of electrodes on the skin that can evaluate the nerve impulses going to the muscle. Past research has demonstrated that changes in neural activation may partially influence muscular force and power development (Behm and Chaouachi, 2011; Marek et al., 2005). Also, the neural inhibition may lessen the ability of skeletal muscle to adjust to high eccentric loads during exercise, which may increase the risk of injury.
Effect of Stretching on Muscular Power, Strength and Speed
Changes in strength and power post-stretching have been reported to range between 5 and 30% (Young and Behm, 2003). Winchester et al. (2008) showed a reduction in 20- and 40-meter sprint time when static stretching followed a general, dynamic warm-up in collegiate track athletes. Needham, Morse and Degens (2009) demonstrated improvements in jumping performance following a dynamic warm-up compared to static stretching in elite, youth soccer players. Young and Behm (2003) compared jumping performance between different warm-up protocols that included a combination of running, jumping and stretching, and found significantly lower jumping performance and force development following static stretching alone. Conversely, other researchers (Handrakis et al., 2010) employed a static-stretching protocol prior to a dynamic balance challenge and jumping tests in 10 middle-age adults, and found an improvement in dynamic balance and no change in jumping performance following static stretching. Curry et al. (2009) found no significant differences in vertical jump and muscular strength between general warm-up (aerobic) activity and static stretching in 24 healthy women.
Findings on the effect of static stretching and sprint performance have been inconsistent. Nelson et al. (2005) reported a significant increase in 20-meter sprint time following an acute bout of static stretching. However, Little and Williams (2006) reported improved 20-meter sprint times in professional soccer players following a warm-up routine that included static stretching.
Muscular strength impairments due to static stretching have been consistently found in research. Nelson, Kokkonen, and Eldredge (2005) showed a 5.7% and 3.6% in one-repetition maximum (1-RM) scores for quadriceps and hamstrings, respectively, following static stretching. Marek et al. (2005) also demonstrated decreases in maximal force following static stretching. Behm, Button, and Butt (2001) showed a 12.2% decrease in isometric strength of the quadriceps following a static stretching protocol.
Running Economy and Energy Expenditure
Compared to muscular power and strength, much less is known about the effects of static stretching on running economy or energy expenditure during exercise. Wilson et al. (2010) measured the energy cost of running following 15 minutes of static stretching in 10 trained runners. They found that energy expenditure, and therefore the energy cost of running, was significantly increased following static stretching. Allison, Bailey and Folland (2008) found an opposite result with running exercise following static stretching. Running economy was not affected by static stretching in 10 trained runners; however, vertical jump and muscular strength were significantly decreased following static stretching. Thus, the mechanisms that are attributed to performance decrements in strength and power are much different than those that influence endurance performance.
After evaluating the current research, it appears that significant reductions in force and power can be elicited by static stretching. However, there are some exceptions to this rule. Two recent review articles have shed more light on why variability in post-stretching responses exists in the literature. Kay and Blazevitch (2012) found that stretching protocols employing 30 seconds or less per stretch elicited no negative effects on strength and power performance. However, decrements in strength and power were found with greater than 60 seconds of stretching. Behm and Chaouachi (2011) uncovered similar results in their review, and concluded that intensity below the level of slight discomfort will not affect muscular performance.
Therefore, the inclusion of pre-exercise stretching and timing of stretching should be considered in a context-specific manner:
- If the goal is to improve range of motion prior to exercise (as with an older adult), then including some static stretching with a dynamic warm-up routine would be appropriate.
- When performing static stretching prior to strength or power performance, it is important to limit the timing of the hold (<30 seconds for sure) and the intensity (below the point of discomfort).
- There is no agreement on whether static stretching prior to endurance exercise is helpful for performance, so caution is recommended. Using a general warm-up that includes dynamic activities may be best.
- There is agreement on the beneficial effects of long-term stretching programs on jump, sprint and strength performance (Kokkonen, Nelson and Eldredge, 2007; Shrier, 2004). Therefore, it is safe to say that decreasing chronic inflexibility should be a main component of a training program.
- Questions still remain regarding the most appropriate dose of stretching prior to exercise performance. In some cases, too much static stretching may hinder performance and in other cases, less static stretching may have no effect and generate little or no improvement in performance.
The Bottom Line: Static stretching before exercise DOES NOT improve exercise performance.
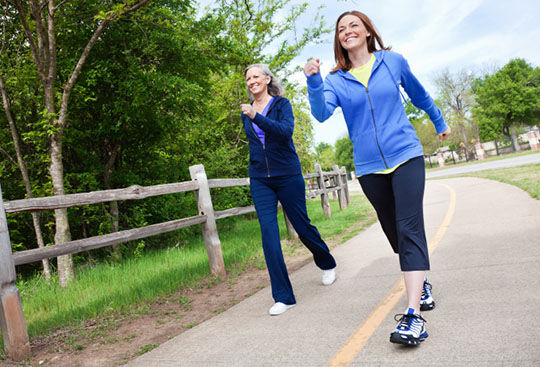
Myth #3: Walking a mile burns as many calories as running a mile.
If the absolute distance covered is the same—1 mile—the total amount of calories burned should be the same, regardless of intensity, right? In fact, many fitness professionals and clients alike conclude that caloric expenditure will not differ between walking and running over this distance.
Not so fast. Researchers (Hall et al., 2004) in the mid-2000s directly measured the energy expenditure of walking a mile (3.2 mph) compared to running a mile (6.3 mph) in a group of 24 healthy participants. The findings? Running had a 40% greater energy cost compared to walking. These findings were comparable whether the exercise was performed on the treadmill or over ground. The authors also reported that the American College of Sports Medicine (ACSM) metabolic equations for running and walking accurately predicted the energy cost of each respective activity.
A simple application of metabolic principles can explain the energy expenditure differences between running and walking. The ACSM metabolic prediction equations for running and walking are presented in the table below (ACSM, 2010). Note that the metabolic cost of running (0.2) is twice that of walking (0.1).
Running Metabolic Equation: VO2 (mL/kg/min) = (0.2 x speed) + (0.9 x speed x grade) + 3.5
Walking Metabolic Equation: VO2 (mL/kg/min) = (0.1 x speed) + (1.8 x speed x grade) + 3.5
Consider the following example:
Leigh (65kg) wants to compare the energy cost of walking 3.0 mph to running 7.5 mph for 1 mile. (Note: Insert speed in meters per minute (m/min); convert mph to m/min by multiplying by 26.8.) Once the VO2 of each activity is determined, use this equation to convert to kcal/min: kcal/min = [(VO2 x body mass)/1000] x 5).
Running Metabolic Equation:
VO2 (mL/kg/min) = (0.2 x 201) + (0.9 x 201 x 0.0%) + 3.5
VO2 = 43.7 mL/kg/min
kcal/min = [(43.7 x 65)/1000] x 5 = 14.2025
Walking Metabolic Equation:
VO2 (mL/kg/min) = (0.1 x 80.4) + (1.8 x 80.4 x 0.0%) + 3.5
VO2 = 11.54 mL/kg/min
kcal/min = [(11.54 x 65)/1000] x 5 = 3.7505
Leigh will expend 113.6 calories running 1 mile (14.2025 kcal/min x 8 min) and 75 calories walking 1 mile (3.7505 kcal/min x 20 min), a 52% difference.
The Bottom Line: Walking a mile DOES NOT burn as many calories as running a mile.
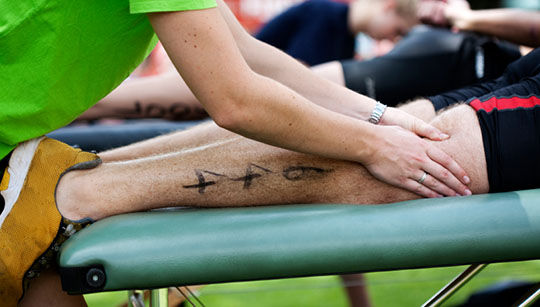
Myth #4: Lactic acid causes acidosis and muscle fatigue during exercise.
The notion that lactic acid or lactate production during exercise is bad has stuck for almost a century. Lactate or lactic acid has been linked to fatigue, the burning sensation felt during exercise, muscle soreness and nausea. The main basis for this myth is a gross misinterpretation of the science of metabolic acidosis and a “guilt-by-association” relationship between fatigue and lactate production. Dr. George Brooks, an international expert on the metabolism of lactate, has referred to the theory of lactic acidosis as “…one of the classic mistakes in the history of science” (Kolata, 2006).
A quick Google search for tips to reduce “lactic acidosis” during exercise reveals some interesting information. Recommendations range from eating bananas to reduce lactic acid levels, drinking lemon juice and staying hydrated to counteract lactic acid, stretching and massage after exercise to reduce soreness caused by lactic acid and taking substances to reduce the formation of lactate. The main problem with these recommendations is that they do not address the main source of metabolic acidosis during exercise.
First, a very brief history lesson: The notion of a lactic acidosis and its link to fatigue can be traced to the Nobel prize−winning work of Otto Meyerhoff and A.V. Hill in 1922 regarding the metabolism of carbohydrate in skeletal muscle (Robergs, Ghiasvand and Parker, 2004). In their collective interpretation, lactic acid was produced in the absence of oxygen during glycolysis and that this metabolism could supply a high amount of energy in a short period of time. During intense exercise, when lactic acid production is high, acidosis increases and fatigue begins to occur. Thus, a cause-and-effect relationship was born that persists to this day. Alternatives to this theory began to be discussed in the 1960s and research reviews questioning the validity of the lactic acidosis concept have since been published (Cairn, 2006; Robergs, Ghiasvand, Parker, 2004; Brooks, 2001). To better understand and refute this common myth, we must take a brief look at the biochemistry of lactate production and acidosis.
Acids and Lactate Production: An acid is a molecule that donates/releases a proton or hydrogen ion (H+) to solution. The concentration of protons determines the pH of a solution (e.g., cytoplasm of cells). However, not all protons come from acids, which is important to remember. The lactate dehydrogenase (LDH) reaction involves the reduction of pyruvate to lactate and the oxidation of the electron carrier molecule nicotinamide adenine dinucleotide (NADH). This is a side reaction to the larger pathway of glycolysis, which ends with the production of pyruvate. The LDH reaction is drawn below:
Pyruvate + NADH+H+ ↔ Lactate + NAD+ (no release of H+)
The concept of “lactic acidosis” states that when lactic acid is produced it immediately is converted to lactate and a free proton is released to solution (lactic acid ↔ lactate + H+). However, in the LDH reaction, the production of lactate from pyruvate does not cause the release of a proton. The biochemical truth is that the LDH reaction involves the addition of two protons to pyruvate to form lactate. In essence, this reaction serves as a buffering mechanism to keep H+ from building up in the cell, not as a contributor to acidosis.
Where does the H+ come from? The current thought is that H+ comes from the accumulation of NADH+H+ during high-intensity exercise, directly from glycolysis and ATP hydrolysis (breakdown of ATP using water). According to Robergs and others, the main source of metabolic acidosis is ATP hydrolysis. When ATP is broken down into ADP + Pi, a proton is released into solution. Thus, the reaction would look like this:
ATP ↔ ADP + Pi + H+
During high-intensity exercise, a significant amount of ATP is produced (in glycolysis and from creatine phosphate use) and broken down for energy. In this state, the protons will begin to accumulate and cause fatigue in the muscle. This process is happening simultaneously with increased lactate production because glycolysis is highly activated. Therefore, the relationship between lactate and proton production is considered to be correlational, not causal.
So, if lactate production isn’t bad, how does it help? Through the LDH reaction, it is clear that lactate does not cause acidosis (Truth #1) and that the molecule NAD+ is formed along with lactate. This molecule is needed during a crucial reaction in glycolysis to keep ATP production going during high-intensity exercise. Thus, if we did not produce lactate, we would have difficulty performing exercise at high intensities (Truth #2) because our capacity to rapidly produce ATP would be reduced. Lastly, lactate has been studied extensively as a source of energy (Truth #3) that can be used directly by slow-twitch fibers during exercise and converted back to glucose in the liver (Brooks, 2001).
How do we apply this information to improve exercise performance? First, we need to understand that the best way to improve endurance and the body’s response to metabolic acidosis during exercise and recovery is to increase the number of mitochondria in our muscle. How do we do that? Research shows us that the most effective way is incorporating occasional bouts of high-intensity interval training into our exercise routine. The inclusion of lower-intensity exercise bouts (so we don’t produce lactic acid) is useful for other reasons, but is less effective at making significant contributions to mitochondrial density. If our muscle is more adapted to rely on aerobic means of ATP production across a range of intensities, we will rely less on anaerobic processes that are related to greater acidosis and premature fatigue.
The Bottom Line: Lactic acid DOES NOT cause acidosis and muscle fatigue.
In part two of this series on exercise myths, we will examine the science behind spot reduction, the fat-burning zone, excess post-exercise oxygen consumption and more.
References
Allison, S.J., Bailey, D.M. and Folland, J.P. (2008). Prolonged static stretching does not influence running economy despite changes in neuromuscular function. Journal of Sports Science, 26, 1489−1495.
American College of Sports Medicine. (2010). ACSM’s Guidelines for Exercise Testing and Prescription (8th ed.). Baltimore, Md.: Lippincott, Williams and Wilkins.
Behm, D.G. and Chaouachi, A. (2011). A review of the acute effects of static and dynamic stretching on performance. European Journal of Applied Physiology, 111, 2633−2651.
Behm, D.G., Button, D.C. and Butt, J.C. (2001). Factors affecting force loss with prolonged stretching. Canadian Journal of Applied Physiology, 26, 261−272.
Brooks, G.A. (2001) Lactate doesn’t necessarily cause fatigue: Why are we surprised? Journal of Physiology, 536, 1.
Cairn, S.P. (2006). Lactic acid and exercise performance: Culprit or friend? Sports Medicine, 36, 279−291.
Curry, B.S. et al. (2009). Acute effects of dynamic stretching, static stretching and light aerobic activity on muscular performance in women. Journal of Strength and Conditioning Research, 23, 1811−1819.
Fletcher, I.M. and Jones, B. (2004). The effect of different warm-up stretch protocols on 20-meter sprint performance in trained rugby union players. Journal of Strength and Conditioning Research, 18, 885−888.
Fredette, D. (2001). Exercise recommendations for flexibility and range of motion. In: Roitman, J. (Ed.) ACSM Resource Manual for Guidelines for Exercise Testing and Prescription (4th ed.). Baltimore, Md.: Lippincott, Williams and Wilkins.
Hall, C. et al. (2004). Energy expenditure of walking and running: Comparison with prediction equations. Medicine and Science in Sports and Exercise, 36, 2128−2134.
Handrakis, J.P. et al. (2010). Static stretching does not impair performance in active middle-aged adults. Journal of Strength and Conditioning Research, 24, 825−830.
Kay, A.D. and Blazevich, A.J. (2012). Effect of acute static stretch on maximal muscle performance: A systematic review. Medicine and Science in Sports and Exercise, 44, 154−164.
Kokkonen, J., Nelson, A.G. and Eldredge, C. (2007). Chronic static stretching improves exercise performance. Medicine and Science in Sports and Exercise, 39, 1825−1831.
Kolata, G. (2006). Lactic acid is not muscles' foe, it's fuel. The New York Times, May 15, 2006.
Little, T. and Williams, A.J. (2006). Effects of differential stretching protocols during warm-ups on high-speed motor capacities in professional soccer players. Journal of Strength and Conditioning Research, 20, 203−207.
Marek, S.M. et al. (2005). Acute effects of static and proprioceptive neuromuscular facilitation stretching on muscle strength and power output. Journal of Athletic Training, 40, 94−103.
McHugh, M.P. and Cosgrave, C.H. (2010). To stretch or not to stretch: The role of stretching in injury prevention and performance. Scandivanian Journal of Medicine and Science in Sports, 20, 2, 169−181.
Needham, R.A., Morse, C.I. and Degens, H. (2009). The acute effect of different warm-up protocols on anaerobic performance in elite youth soccer players. Journal of Strength and Conditioning Research, 23, 2614−2620.
Nelson, A.G. et al. (2005). Acute effects of passive muscle stretching on sprint performance. Journal of Sports Sciences, 23, 5, 449−454.
Nelson, A.G., Kokkonen, J. and Eldredge, C. (2005). Strength inhibition following an acute stretch is not limited to novice stretchers. Research Quarterly: Exercise and Sport, 76, 4, 500−506.
Pope, R.P. et al. (2000). A randomized trial of preexercise stretching for prevention of lower-limb injury. Medicine and Science in Sports and Exercise, 32, 271−277.
Robergs, R.A., Ghiasvand, F. and Parker, D. (2004). Biochemistry of exercise-induced metabolic acidosis. American Journal of Physiology − Regulatory, Integrative and Comparative Physiology, 287, 2, R502−R516.
Shrier, I. (2004). Does stretching improve performance? A systematic and critical review of the literature. Clinical Journal of SportsMedicine, 14, 5, 267−273.
Shrier, I. (1999). Stretching before exercise does not reduce the risk of local muscle injury: A critical review of the clinical and basic science literature. Clinical Journal of Sports Medicine, 9, 221−227.
Wilson, J.M. et al. (2010). Effects of static stretching on energy cost and running endurance performance. Journal of Strength and Conditioning Research, 24, 2274−2279.
Winchester, J.B. et al. (2008). Static stretching impairs sprint performance in collegiate track and field athletes. Journal of Strength and Conditioning Research, 22, 1, 13−19.
Witvrouw, E. et al. (2004). Stretching and injury prevention: An obscure relationship. Sports Medicine, 34, 443−449.
Young, W.B. and Behm, D.G. (2003). Effects of running, static stretching and practice jumps on explosive force production and jumping performance. Journal of Sports Medicine and Physical Fitness, 43, 21−27.
_____________________________________________________________________________
Lance C. Dalleck, Ph.D., ACSM-RCEP, is academic coordinator of the Cardiac Rehabilitation postgraduate program at the University of Auckland in New Zealand. His research interests include improving exercise performance and health outcomes through evidence-based practice, quantifying the energy expenditure of outdoor and non-traditional types of physical activity, and studying historical perspectives in health, fitness and exercise physiology.
Jeffrey M. Janot, Ph.D., ACSM-CES, is an associate professor in Kinesiology at the University of Wisconsin−Eau Claire. His current research focuses on various aspects of sports physiology and strength and conditioning, cardiovascular physiology, and enhancing functional abilities of younger and older adults.